Unveiling the Intricacies of the RNA Later Recipe: A Molecular Preservation Technique
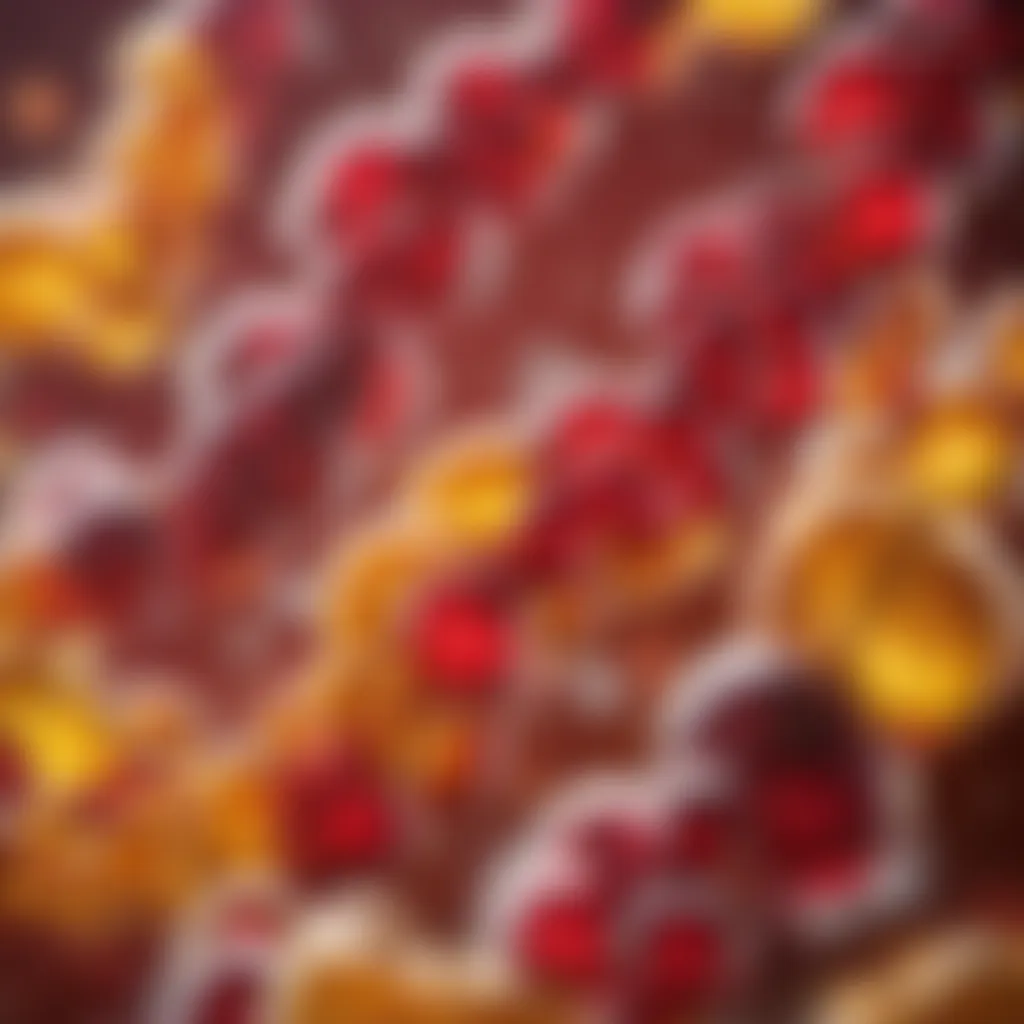
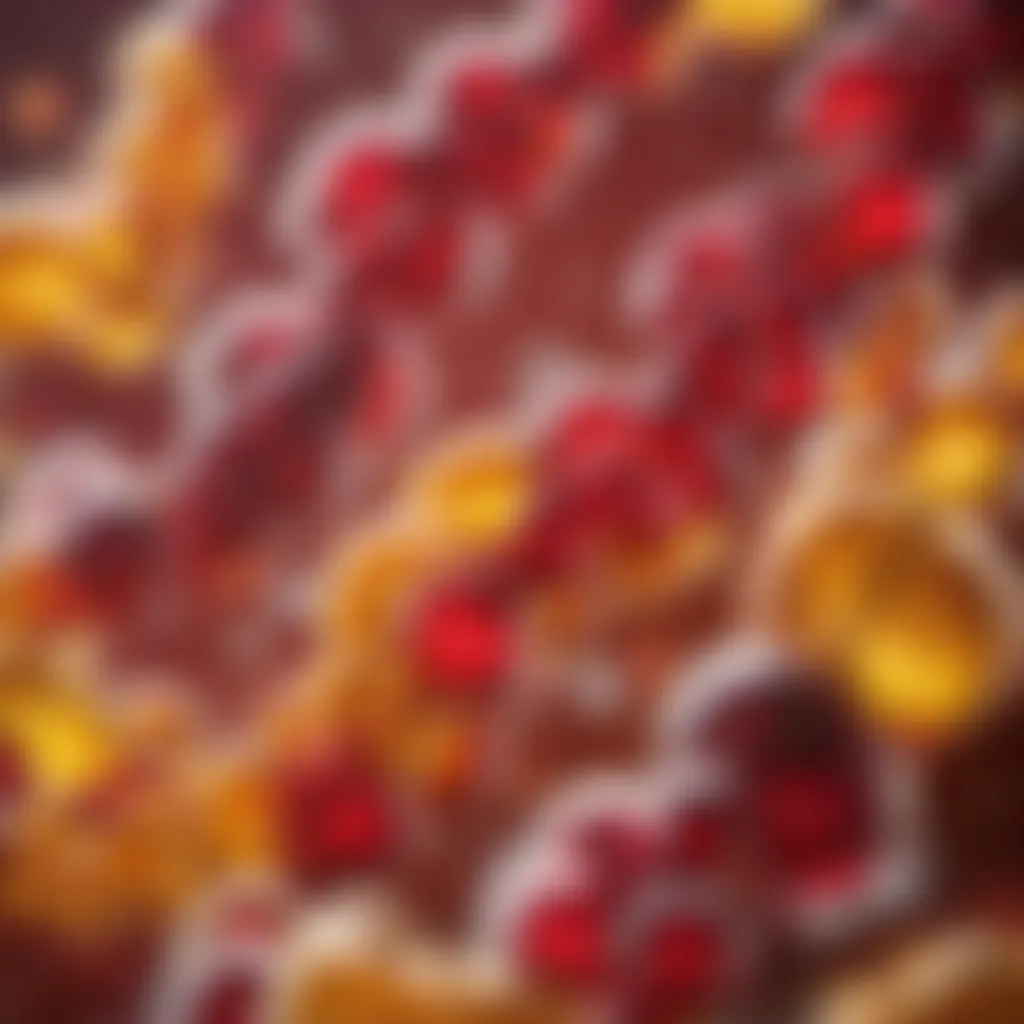
Ingredients:
- RNA Later solution - 500ml
- Ethanol (95-100%) - 100ml
- Centrifuge tubes
- Microcentrifuge
- -20°C freezer
Detailed Instructions:
- Begin by preparing the RNA Later solution in a clean, sterile container.
- Carefully measure 500ml of RNA Later solution and transfer it to the container.
- Add 100ml of ethanol (95-100%) to the mixture slowly, ensuring thorough mixing.
- Label the centrifuge tubes for later use.
- Gently pour the RNA Later solution into the labeled tubes.
- Close the tubes securely and proceed to centrifuge them at 4000 × g for 10 minutes.
- Place the tubes in a -20°C freezer for storage.
Technical Aspects:
- Ensure all equipment is properly sanitized to avoid contamination.
- Maintain a sterile environment throughout the preparation process.
- Centrifuge the tubes at 4000 × g for adequate precipitation of RNA.
Sequential Steps:
- Prepare the RNA Later solution as instructed above.
- Transfer the solution into labeled centrifuge tubes and centrifuge at 4000 × g.
- Store the tubes in a -20°C freezer for preservation.
Troubleshooting Tips:
- If RNA precipitation is incomplete, increase centrifugation time.
- Use caution when handling ethanol to prevent exposure.
- Verify that the freezer maintains a consistent temperature for optimal storage conditions.
Introduction to RNA Later
In the realm of molecular biology and scientific research, understanding the critical role of RNA Later is paramount. The Introduction sets the stage for delving into the intricate details of this crucial technique in RNA sample preservation for research purposes. By laying the foundation with a comprehensive overview of RNA Later, readers will be equipped to grasp the complexities and nuances of this fundamental scientific process.
Definition and Purpose
Overview of RNA Later Solution
The Overview of RNA Later Solution encapsulates the essence of this pivotal technique. This section serves as the gateway to comprehending the science behind RNA Later's functionality, emphasizing its unparalleled ability to maintain RNA integrity and stability. Its key characteristic lies in its capacity to preserve RNA samples by inhibiting degradation pathways effectively. This characteristic makes RNA Later a staple choice for researchers seeking to safeguard the quality of their RNA samples, ensuring the reliability and accuracy of subsequent molecular analyses. The unique feature of RNA Later lies in its seamless integration into sample collection protocols, offering a convenient and efficient solution for researchers in need of robust RNA stabilization methods.
Importance in RNA Stabilization
The importance of RNA Stabilization cannot be overstated in the realm of molecular biology and scientific research. This aspect of RNA Later plays a pivotal role in safeguarding the structural integrity of RNA molecules, thereby enabling accurate gene expression analysis and transcriptomic profiling. Its key characteristic lies in its ability to prevent RNA degradation, ensuring that RNA samples remain intact and viable for downstream molecular studies. The crucial advantage of RNA Stabilization provided by RNA Later is its capacity to maintain RNA quality over extended storage periods, a critical aspect for long-term research projects. Researchers benefit from the dependable RNA stabilization offered by RNA Later, minimizing variability and maximizing the reliability of experimental results.
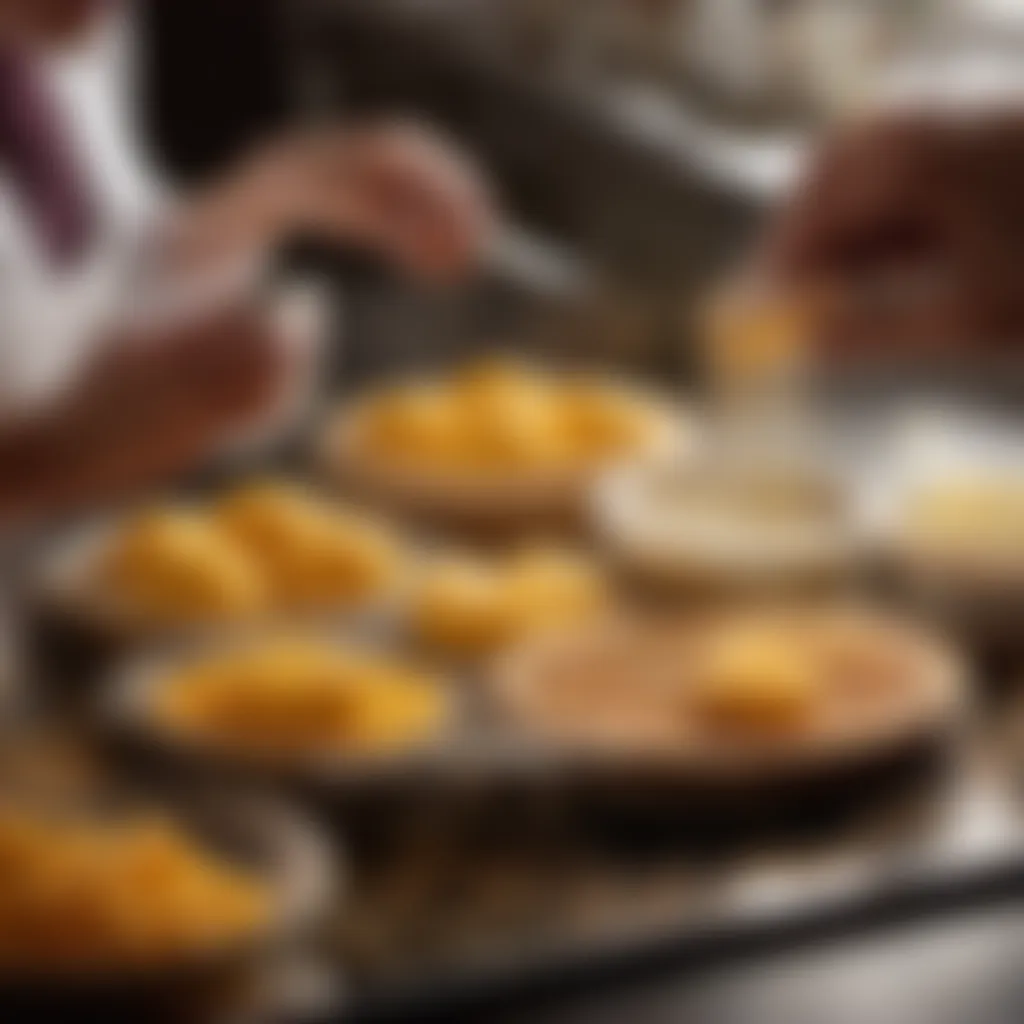
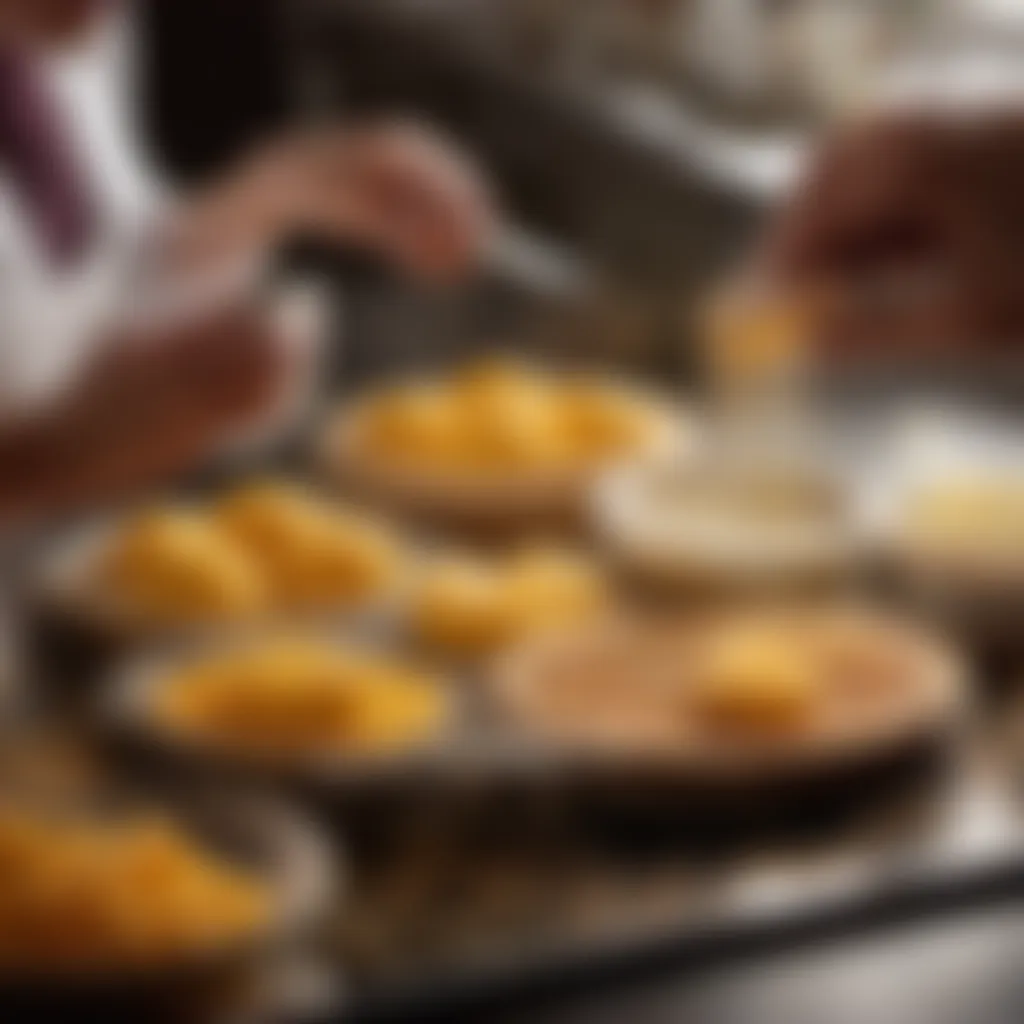
Historical Background
Origins of RNA Later Development
Exploring the Origins of RNA Later Development unveils the fascinating journey that led to the creation of this revolutionary technique. This segment delves into the innovative minds and scientific endeavors that culminated in the inception of RNA Later as a pioneering RNA preservation method. The key characteristic of the Origins of RNA Later Development lies in its disruptive impact on traditional sample preservation techniques, offering a paradigm shift towards more effective and reliable RNA stabilization approaches. The unique feature of this development lies in its ability to address longstanding challenges in RNA sample preservation, revolutionizing the way researchers store and protect valuable genetic material for scientific exploration.
Evolution of Preservation Techniques
The Evolution of Preservation Techniques traces the developmental trajectory of RNA stabilization methods, highlighting the progressive evolution towards more advanced and sophisticated approaches. This section underscores the continuous refinement and enhancement of preservation strategies over time, with RNA Later emerging as a cutting-edge solution in the landscape of molecular biology. Its key characteristic lies in its adaptability and efficiency in preserving RNA samples across diverse research settings, catering to the evolving needs of the scientific community. The unique feature of this evolution lies in its transformative impact on research practices, shaping the way scientists approach RNA sample handling and storage, ushering in a new era of precision and reliability in molecular investigations.
Composition and Mechanism
Exploring the intricate details of the composition and mechanism of the RNA Later recipe is essential to understanding its pivotal role in preserving RNA samples for scientific research. This section delves deep into the fundamental elements that make up RNA Later, shedding light on its mechanisms and benefits within the realm of molecular biology. By dissecting the composition and mechanism of this solution, researchers and scientists can appreciate the intricacies involved in maintaining RNA integrity for various applications in the field.
Key Ingredients
GuSCN
GuSCN, or Guanidinium thiocyanate, stands out as a crucial component in the RNA Later recipe, playing a significant role in RNA stabilization and protection. Its key characteristic lies in its ability to disrupt cellular structures and deactivate RNases, thereby preserving RNA integrity effectively. The unique feature of GuSCN is its strong chaotropic properties, which aid in denaturing proteins and ensuring RNA remains intact during storage and transportation. Despite its potent preservation capabilities, GuSCN may pose certain challenges, such as potential cytotoxicity and difficulty in handling, which should be carefully considered in research settings.
Ethanol
Ethanol serves as another vital ingredient in the RNA Later recipe due to its ability to precipitate nucleic acids efficiently. Its key characteristic lies in its ability to promote RNA aggregation and precipitation, aiding in the separation of RNA from other cellular components. Ethanol is a popular choice for RNA stabilization due to its cost-effectiveness and compatibility with downstream analysis techniques. However, it is crucial to note that ethanol may lead to RNA degradation if not used in optimal concentrations, underscoring the importance of precise ethanol dilutions in RNA Later protocols.
Preservation Process
Cellular RNA Protection
The aspect of cellular RNA protection plays a critical role in the preservation process facilitated by RNA Later. By shielding RNA molecules from degradation and maintaining their structural integrity within cells, cellular RNA protection ensures the longevity of RNA samples for downstream analyses. The key characteristic of this process lies in its ability to prevent enzymatic degradation and preserve RNA in a quiescent state, ready for gene expression studies and other molecular analyses. While offering significant advantages in RNA stability, cellular RNA protection requires careful handling to avoid contamination and oxidative damage during sample collection and storage.
Inhibition of Degradation Pathways
Inhibiting degradation pathways is a key mechanism employed by RNA Later to safeguard RNA samples from enzymatic breakdown and degradation processes. By interfering with nucleases and degradation enzymes, this process ensures that RNA molecules remain intact and viable for extended periods. The unique feature of degradation pathway inhibition lies in its ability to maintain RNA integrity even in challenging environmental conditions, making it an indispensable aspect of effective RNA preservation strategies. However, it is crucial to be mindful of potential drawbacks, such as reduced enzymatic activity in certain downstream applications, when employing this preservation mechanism.
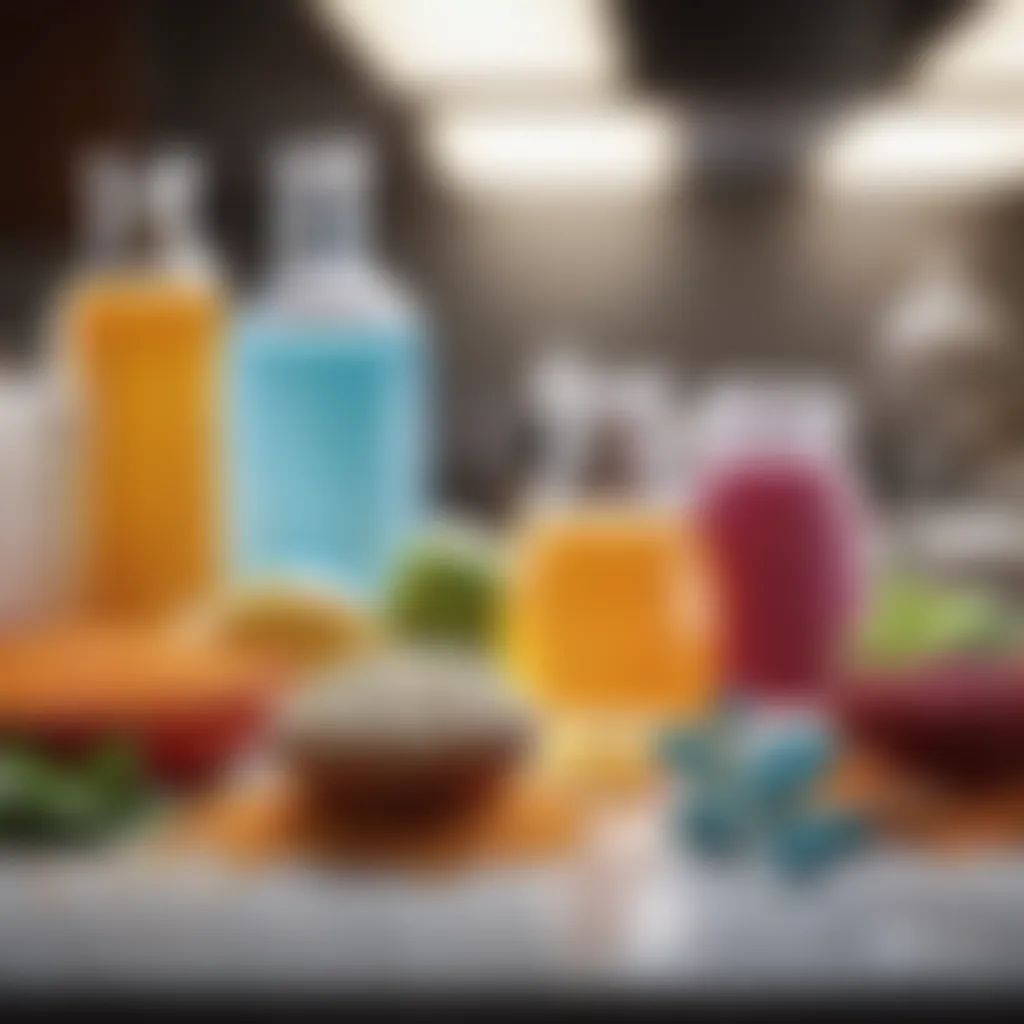
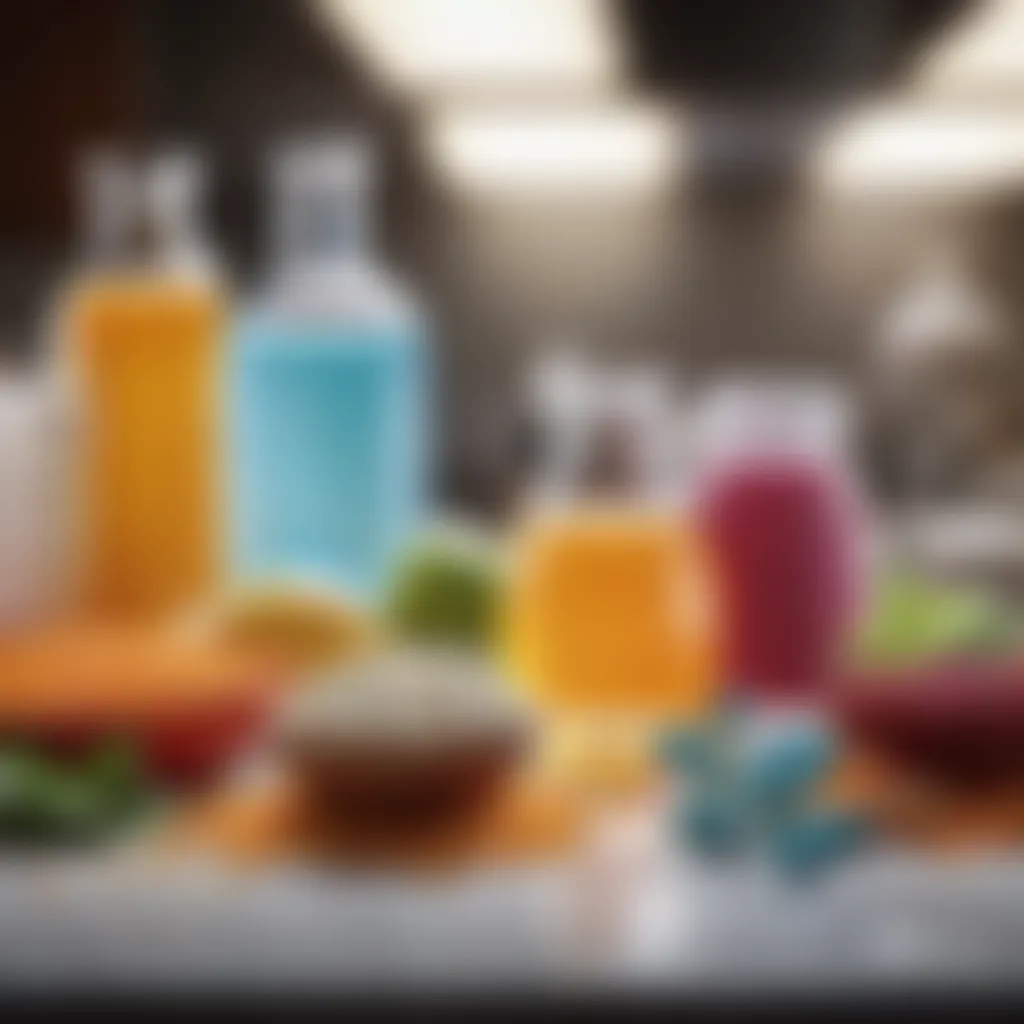
Applications in Research
In this article, the section 'Applications in Research' serves as a pivotal component shedding light on the practical implementations of the RNA Later recipe within the realm of scientific inquiry. This segment meticulously conveys the significance of leveraging RNA Later in diverse research avenues, underlining its pivotal role in molecular biology studies and diagnostic advancements. By exploring the multifaceted applications of RNA Later, readers are primed to grasp the nuanced ways in which this preservation technique fuels groundbreaking discoveries and transformative innovations. The section 'Applications in Research' provides a comprehensive overview of how RNA Later not only safeguards genetic material but also paves the way for cutting-edge research endeavors.
Molecular Biology Studies
Gene Expression Analysis
Gene expression analysis represents a cornerstone within molecular biology studies, enabling researchers to unravel the intricacies of genetic activity. This facet of analysis delves deep into understanding how genes function, offering invaluable insights into their regulation and manifestation. By scrutinizing gene expression patterns, scientists can decipher cellular processes, identify disease mechanisms, and unveil therapeutic targets. Gene expression analysis emerges as a powerful tool in elucidating the molecular underpinnings of biological phenomena, making it a fundamental aspect of this article. Its capability to unveil critical genetic information and provide a molecular blueprint signifies its indispensable role in advancing scientific knowledge. Despite its merits, gene expression analysis may pose challenges related to data interpretation and standardization, underscoring the need for meticulous experimental design and rigorous validation.
Transcriptomic Profiling
Transcriptomic profiling stands at the forefront of deciphering the dynamic landscape of gene transcription within biological systems. This specialized analysis elucidates the complete set of transcripts present in a cell or tissue, offering a comprehensive view of gene expression patterns. By unraveling the transcriptome, researchers can decipher intricate regulatory networks, pinpoint biomarkers, and unravel disease signatures. Transcriptomic profiling emerges as a preferred choice in this article due to its ability to capture the entirety of RNA transcripts, providing a holistic perspective on cellular behavior. The unique feature of transcriptomic profiling lies in its capacity to generate vast amounts of data, enabling in-depth analyses of cellular processes. However, managing and analyzing such voluminous datasets pose logistical challenges, necessitating robust bioinformatic pipelines and computational expertise.
Diagnostic and Therapeutic Advancements
Disease Biomarker Identification
The process of disease biomarker identification stands as a pivotal mechanism in stratifying patient populations, assessing disease progression, and enhancing diagnostic accuracy. This specialized approach involves pinpointing molecular signatures that correlate with specific diseases, offering personalized insights into individual health profiles. Disease biomarker identification plays a pivotal role in precision medicine by tailoring treatments to patient-specific requirements and predicting disease outcomes. Notably, this aspect of diagnostics represents a crucial element in the article, emphasizing its role in elucidating disease mechanisms and driving therapeutic innovations. Despite its promise, disease biomarker identification encounters challenges in terms of biomarker validation, biomarker heterogeneity, and clinical utility, emphasizing the need for stringent validation and rigorous clinical trials.
Precision Medicine Innovations
Precision medicine innovations epitomize the pinnacle of tailored healthcare approaches, leveraging genomic insights to customize treatment strategies. This progressive field integrates genomics, molecular profiling, and data analytics to deliver personalized therapies that address individual genetic predispositions. Precision medicine innovations emerge as a preferred focus in this article due to their transformative potential in revolutionizing healthcare delivery and therapeutic outcomes. The unique feature of precision medicine lies in its individualized approach to patient care, wherein treatments are tailored based on genetic markers and molecular profiles, enhancing treatment efficacy and patient outcomes. However, the adoption of precision medicine faces challenges related to data privacy, regulatory frameworks, and interprofessional collaboration, necessitating a comprehensive approach towards implementation and integration into clinical practice.
Optimizing RNA Later Usage
In the realm of molecular biology research, optimizing RNA Later usage stands as a pivotal facet that demands meticulous attention and adherence to precise protocols. The overarching goal of this section within the article is to delve deep into the methodologies, practices, and considerations that can significantly enhance the efficacy and reliability of RNA preservation using RNA Later solution. By emphasizing the importance of optimizing RNA Later usage, researchers and scientists gain invaluable insights into maximizing the stability and integrity of RNA samples for downstream applications.
Best Practices
Storage Conditions
Navigating the intricate world of RNA preservation, zeroing in on optimal storage conditions is paramount in ensuring the longevity and quality of RNA samples. The precise control of temperature, humidity, and light exposure plays a crucial role in maintaining RNA stability within the RNA Later solution. The unique characteristic of storage conditions lies in their ability to shield RNA molecules from degradation and ensure that the integrity of genetic material remains intact. By implementing appropriate storage conditions, researchers can mitigate the risk of RNA degradation and preserve the sample for extended periods, bolstering the reliability of downstream analyses and experiments.
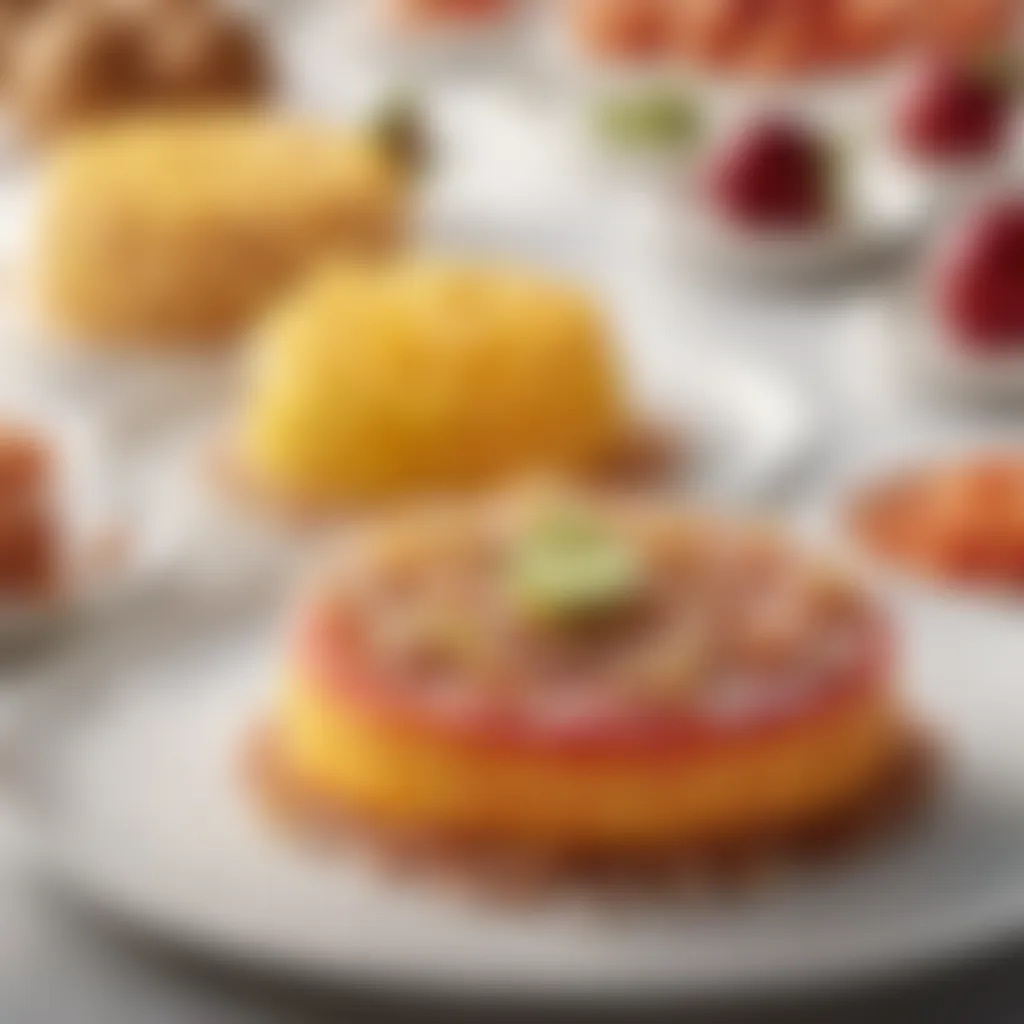
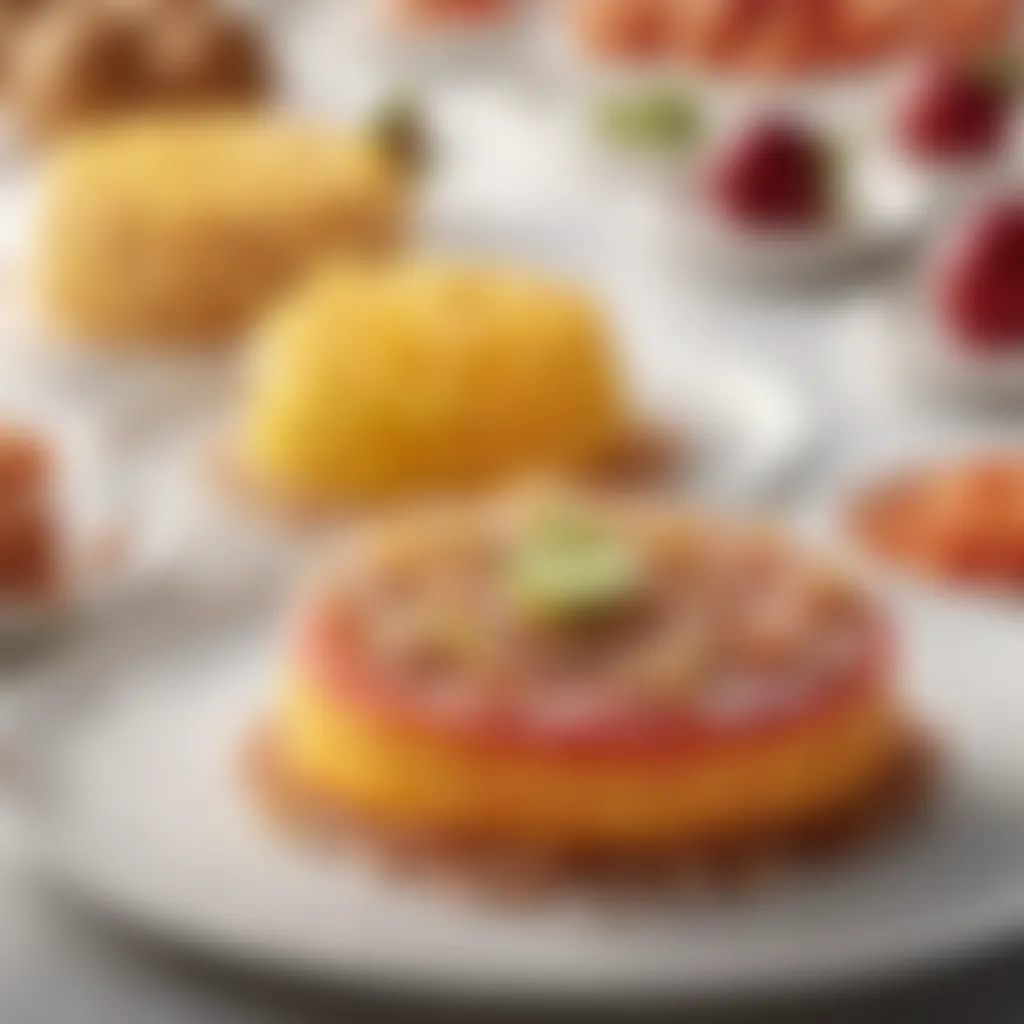
Sample Collection Protocols
When it comes to optimizing RNA Later usage, meticulous attention to sample collection protocols is indispensable. Efficient and thorough sample collection procedures ensure the retrieval of high-quality RNA specimens, laying the foundation for successful preservation using RNA Later solution. The key characteristic of sample collection protocols lies in their ability to minimize RNA degradation risks during collection, handling, and processing. By adhering to standardized sample collection protocols, researchers can enhance the integrity of RNA samples, thus optimizing the efficacy of RNA Later preservation. Understanding the nuances of sample collection protocols is crucial for researchers aiming to extract accurate and reliable data from preserved RNA samples.
Common Pitfalls to Avoid
Contamination Risks
Diving into the intricacies of RNA later usage unveils the critical consideration of contamination risks that pose a threat to the integrity of RNA samples. Contamination can arise from various sources, including environmental factors, equipment, or improper handling procedures. The key characteristic of contamination risks lies in their potential to compromise the quality and validity of RNA samples, leading to skewed results and inaccurate data interpretation. By acknowledging and mitigating contamination risks through stringent cleanliness protocols and rigorous validation methods, researchers can safeguard the integrity of RNA samples, thereby ensuring the reliability of subsequent molecular analyses.
Improper Handling Effects
In the landscape of RNA preservation, improper handling effects emerge as a significant pitfall that researchers must navigate with precision and care. Improper handling of RNA samples can result in RNA degradation, cross-contamination, or sample loss, jeopardizing the reliability and reproducibility of research outcomes. The unique feature of improper handling effects lies in their potential to introduce errors and inconsistencies in molecular analyses, compromising the scientific rigor of research findings. Researchers must prioritize proper training, adherence to standard handling procedures, and the implementation of quality control measures to circumvent the detrimental impact of improper handling effects on RNA sample integrity.
Future Trends and Innovations
In the realm of scientific research, keeping a pulse on future trends and innovations is paramount to staying ahead in the ever-evolving field of molecular biology. This section of the article delves deep into the significance of anticipating and adapting to emerging technologies that shape the landscape of RNA preservation and analysis.
Emerging Technologies
RNA Later Alternatives
Exploring RNA Later alternatives unveils a revolutionary shift in preserving RNA samples for research. These alternatives offer a progressive approach to RNA stabilization, providing researchers with diverse options to enhance sample quality and longevity. One key characteristic of RNA Later alternatives lies in their versatility and adaptability to various experimental needs. Their flexibility in application makes them a preferred choice for researchers seeking tailored solutions for RNA preservation. Additionally, the unique feature of RNA Later alternatives lies in their potential to streamline workflows and improve RNA isolation efficiencies. While these alternatives exhibit many advantages, such as promoting sample integrity and simplifying preservation protocols, it is essential to carefully assess their compatibility with specific research objectives.
Enhanced Preservation Methods
The discussion on enhanced preservation methods brings to light cutting-edge techniques that redefine the standards of sample storage and RNA protection. Enhanced preservation methods are characterized by their advanced mechanisms designed to optimize RNA stability and integrity over extended periods. Their key characteristic lies in the precision and efficacy with which they inhibit degradation pathways, ensuring the preservation of RNA quality for downstream analyses. These methods stand out as a beneficial choice for researchers aiming to elevate the reliability and reproducibility of their experimental results. Moreover, the unique feature of enhanced preservation methods is their ability to offer unmatched protection against environmental factors that could compromise RNA integrity. While these methods boast significant advantages in safeguarding RNA samples against degradation, it is crucial to consider the potential limitations they may pose regarding certain experimental requirements.
Potential Research Frontiers
Exploring potential research frontiers opens doors to exciting possibilities in the realm of RNA sequencing and single-cell analysis. These frontiers represent novel avenues for delving deeper into the intricacies of molecular biology and expanding the horizons of scientific exploration.
RNA Sequencing Applications
The discussion on RNA sequencing applications illuminates a transformative approach to unraveling the complexities of gene expression patterns and transcriptomic profiles. Characterized by their high-throughput capabilities, RNA sequencing applications offer a comprehensive insight into the dynamic nature of RNA populations within biological systems. Their key characteristic lies in the robustness and sensitivity with which they capture gene expression dynamics, making them a popular choice for researchers seeking in-depth molecular insights. The unique feature of RNA sequencing applications is their capacity to identify rare RNA species and detect subtle variations in gene expression levels with unparalleled precision. While these applications hold immense advantages in revolutionizing mRNA analysis and uncovering novel RNA biomarkers, researchers must carefully consider the scalability and data analysis challenges associated with high-throughput sequencing techniques.
Single-Cell Analysis Developments
Delving into single-cell analysis developments unveils groundbreaking methodologies that revolutionize the study of individual cells within complex biological systems. These developments empower researchers to dissect cellular heterogeneity and unravel the intricacies of cellular functions at a single-cell resolution. The key characteristic of single-cell analysis developments lies in their ability to capture cellular diversity and dynamics with unprecedented detail, enabling a deeper understanding of cellular processes at the molecular level. Their unique feature lies in the potential to uncover rare cell populations and dissect cellular interactions in intricate biological contexts. While these developments offer unparalleled advantages in deciphering cellular heterogeneity and elucidating cellular pathways, researchers should be mindful of the technological constraints and experimental complexities associated with single-cell analysis techniques.