Exploring the Complexities of Water Freezing
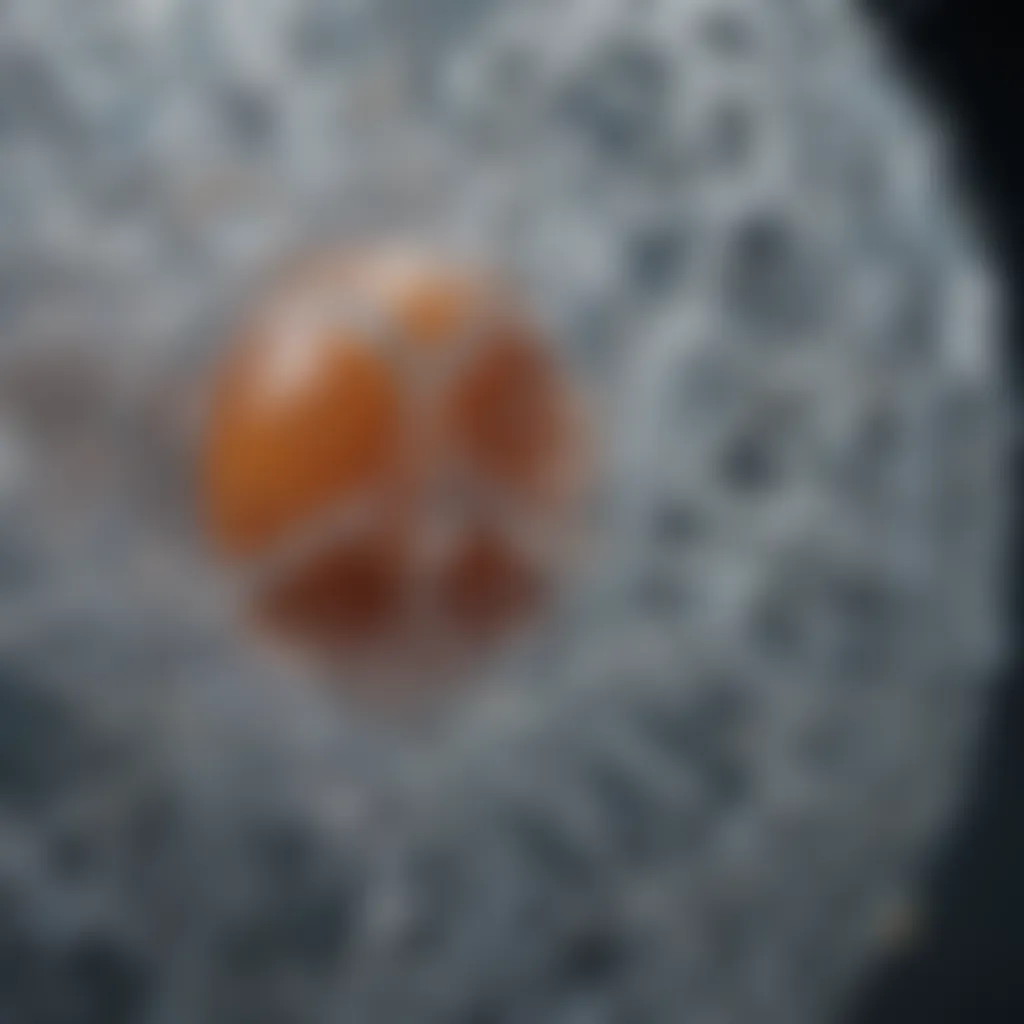
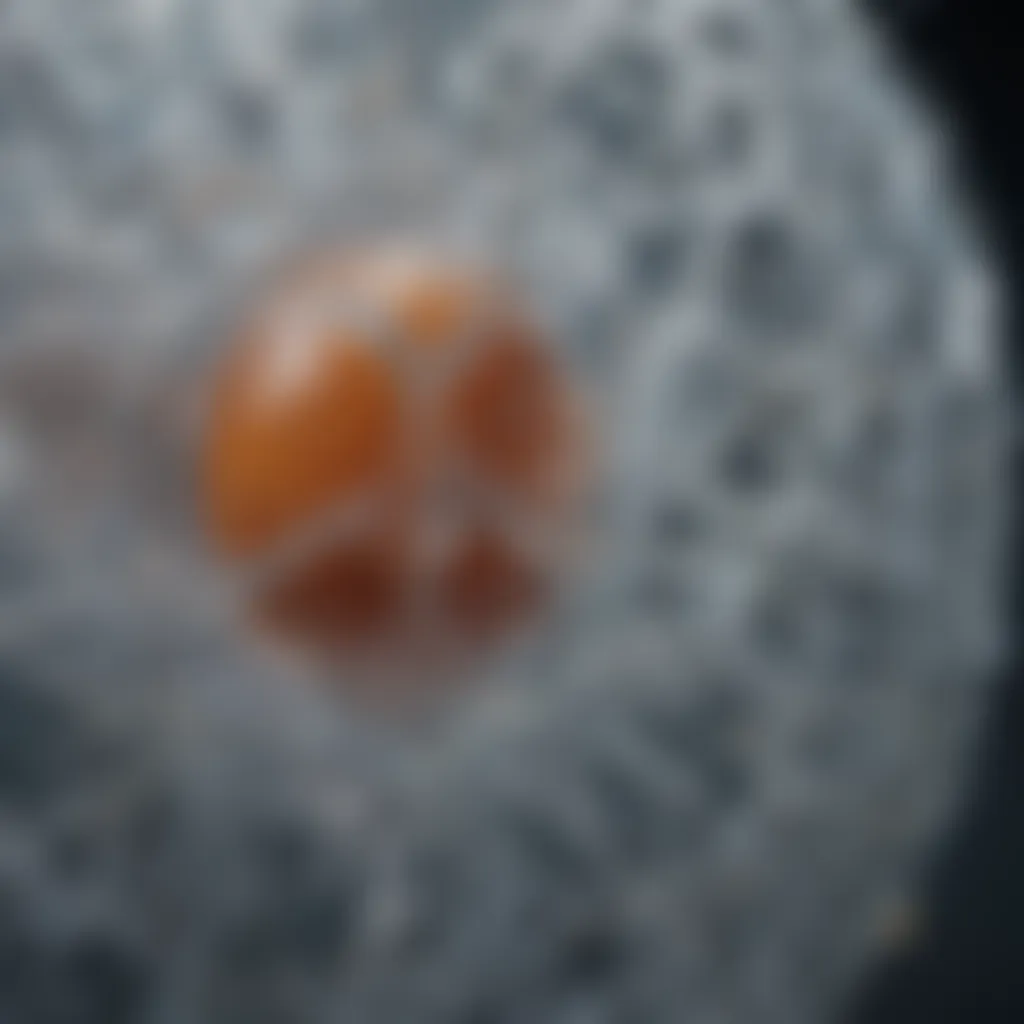
Intro
Water, often taken for granted, displays fascinating behavior when it undergoes the freezing process. The transition from liquid to solid not only affects the water itself but also has far-reaching implications in various environments, from the natural world to industrial applications. Understanding this process requires a closer look at the molecular interactions, thermodynamic principles, and the unique attributes of water that come into play. This investigation aims to dissect these intricacies and elucidate how they shape both our daily lives and broader environmental phenomena.
By peeling back the layers of scientific mechanisms, we can gain a deeper appreciation for the role of water in our ecosystem and its broader applications. From freezing rain to the formation of ice on lakes, the popping sound of ice cubes in a drink reminds us of the mysteries behind this simple yet profound transformation.
As we journey deeper into the heart of freezing, we will explore not just the fundamental principles, but also how they manifest physically and chemically, helping us connect with the world around us more meaningfully.
Understanding Phase Changes
Understanding the concept of phase changes is crucial to grasping the complexities of how water freezes. Phase changes, or transitions between states of matter such as solid, liquid, and gas, play a vital role in various natural and industrial processes. Knowing how and why these changes occur can inform better practices in everything from cooking to climate science. This section lays the groundwork for the following discussions by examining essential concepts related to phase changes in water, particularly its freezing behavior.
Definition of Phase Changes
Phase changes refer to the transformation of a substance from one state of matter to another as a result of changes in temperature or pressure. Common examples include the melting of ice into water or the evaporation of water into steam. At the molecular level, these transitions involve alterations in the arrangement and energy of particles. For instance, in solid ice, molecules are structured in a rigid lattice, whereas in liquid water, they are more loosely arranged, allowing for mobility.
To sum it up, phase changes occur when energy is added or withdrawn from a substance:
- Melting: solid to liquid (e.g., ice to water)
- Freezing: liquid to solid (e.g., water to ice)
- Evaporation: liquid to gas (e.g., water to vapor)
- Condensation: gas to liquid (e.g., vapor to water)
- Sublimation: solid to gas without becoming liquid (e.g., dry ice)
Understanding these transitions helps to uncover the mechanisms behind freezing and can lead to practical applications such as preserving food, engineering, and environmental studies.
Role of Temperature and Pressure
Temperature and pressure significantly influence phase changes, and freezing is no exception. As temperatures drop, motion among molecules begins to slow down. In terms of practical examples, as you chill a pot of water on the stove, you notice steam rising at first. However, as you reduce the heat further, water eventually reaches a point where it freezes into ice.
Pressure also affects freezing - consider when you’re at high altitudes, where the atmospheric pressure is lower. At such heights, water may freeze at a temperature above the typical freezing point of 0°C (32°F). Conversely, applying pressure increases the melting point of ice, which is why it is harder to find ice in a pressurized environment, such as deep below the ocean.
Understanding how temperature and pressure interact with phase changes provides meaningful insights into weather behavior, food science, and eco-system dynamics.
In summary, grasping the relationship between temperature, pressure, and phase changes shapes how we understand the freezing of water, revealing nuances that affect both our natural world and everyday applications.
Thermodynamics of Freezing
Understanding the thermodynamics of freezing is essential, not just for scientists but also for those of us who engage with water in our daily lives, such as in cooking or keeping our beverages cool. When contemplating how water transitions from a liquid to solid state, one cannot overlook the behind-the-scenes workings of thermodynamic laws and heat transfer mechanisms. These principles govern everything from the shape of an icicle to the occurrence of frost on your window.
Laws of Thermodynamics
At the very heart of thermodynamics lie its foundational laws, which illustrate how energy moves and transforms.
- First Law (Law of Energy Conservation): This law posits that energy cannot be created or destroyed; it can only transition from one form to another. When water freezes, the energy it retains diminishes as it expels heat into the surrounding environment. This idea is pivotal in understanding why lakes freeze from the top down—instead of top-up that might defy nature’s way.
- Second Law: This law introduces the concept of entropy, which can be seen as a measure of disorder within a system. As water freezes into ice, the movement of molecules slows down, leading them to settle into a structured crystalline lattice. This transformation reduces the entropy, indicating a more ordered state of existence for the water molecules, which might seem counterintiuitive at first glance.
- Third Law: The third law discusses how absolute zero, which is -273.15°C, is unattainable. As water approaches freezing temperatures, molecular motion decreases significantly, but it never halts altogether. It provides a fascinating insight into the lifelike characteristics of ice even on the coldest days.
Through these laws, it becomes clear that freezing is a stage in a broader dialogue between energy and matter, a dance that both shapes our physical world and our understanding of it.
Heat Transfer Mechanisms
Heat transfer is a crucial element when delving into the freezing process. The mechanisms are generally categorized into three modes: conduction, convection, and radiation. Each plays a distinct role in how water cools and eventually freezes.
- Conduction: This is the direct transfer of heat through materials. For example, if you leave a glass of water on a cold countertop, the heat from the water will transfer to the cooler surface, leading to a drop in the water's temperature. This method is why ice cubes placed in a drink start to melt as they absorb warmth from the liquid.
- Convection: This process involves the movement of fluids and their heat content. Ocean currents, for instance, are constantly moving warmer water, which can profoundly influence how ice forms on the sea surface. Cooler, denser water sinks while warmer water rises, continually facilitating a balance that could affect freezing rates.
- Radiation: Even though it seems less direct, radiation plays a role too. Heat emitted from the sun or nearby objects warms water bodies, but excess heat lost to the atmosphere can deter freezing, especially at night when no sunlight warms the surface.
It’s worth noting that the environment plays a significant role in how heat is transferred. Factors like wind chill, humidity, and barometric pressure can all impact how quickly water reaches freezing temperatures.
"The freezing of water is not merely a physical phenomenon but a structured conversation of energy and its surroundings."
In exploring the thermodynamics of freezing, we appreciate the balancing act of various elements that all contribute to the enchanting yet complex process of ice formation. The knowledge gained goes beyond mere academic interest; it can deeply affect how we interact with water in our everyday lives, from household freezes to global climate considerations.
Molecular Structure of Water
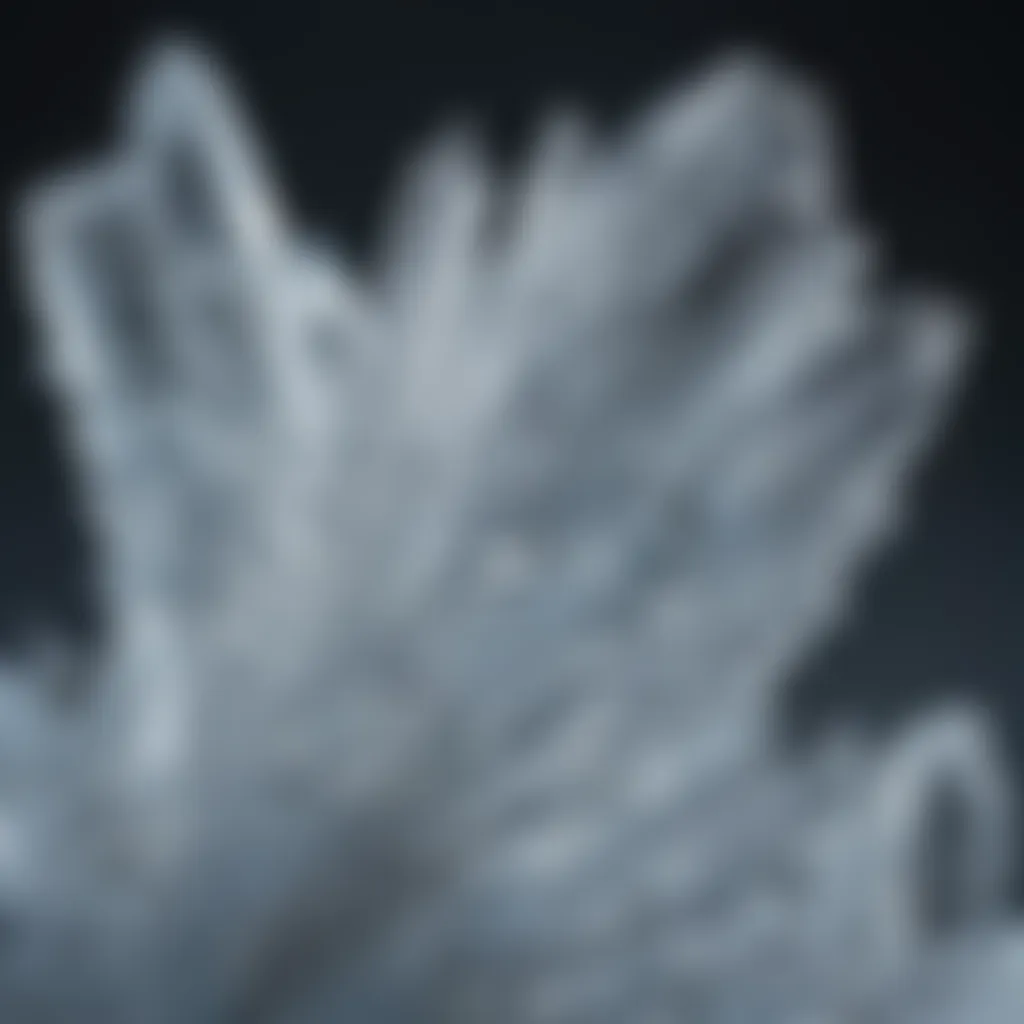
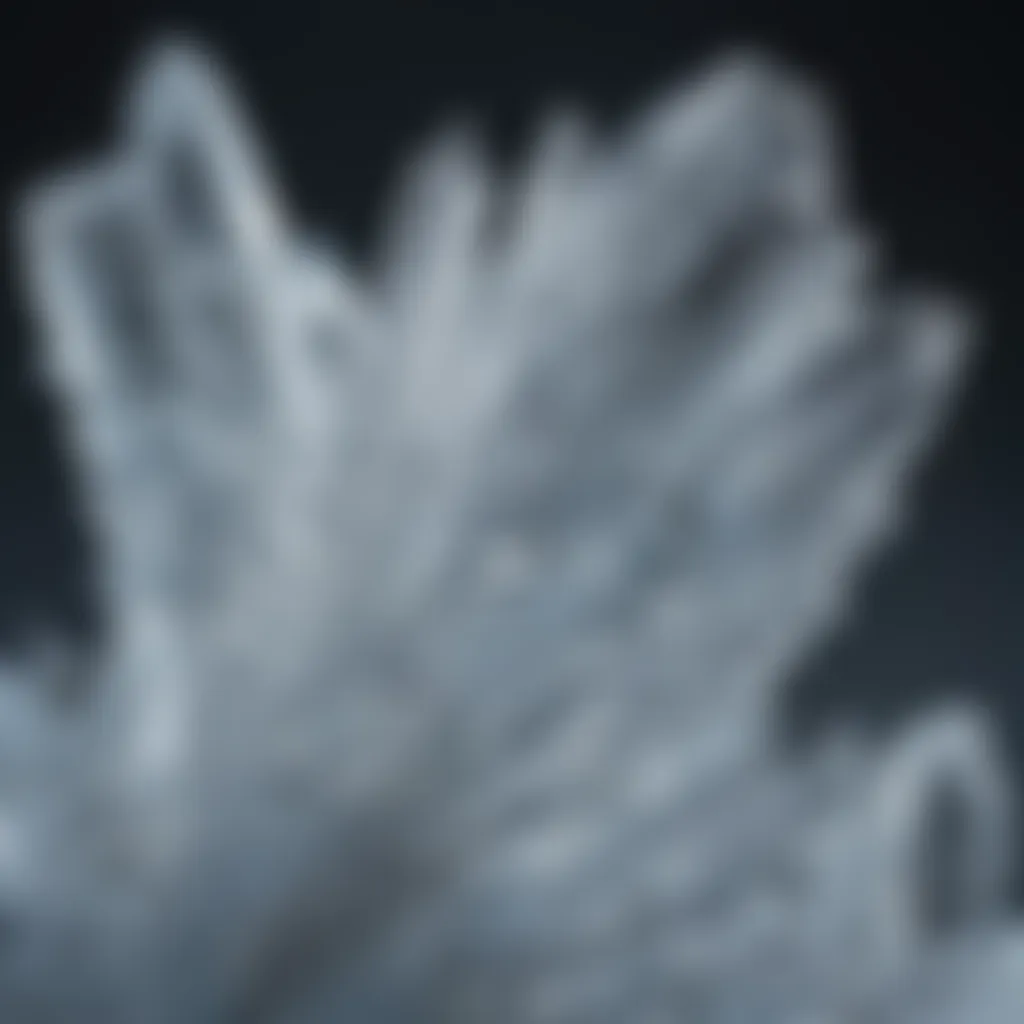
Understanding the molecular structure of water is fundamental to grasping how freezing occurs. Water, composed of two hydrogen atoms bonded to one oxygen atom, exhibits unique characteristics that set it apart from other substances. These characteristics not only influence the freezing process but also impact various physical and biological properties essential for life.
Hydrogen Bonding in Water
Water molecules are held together by hydrogen bonds, a type of weak attraction between the hydrogen atom of one molecule and the oxygen atom of another. This bonding works like a chain, where each water molecule is linked to its neighbors. The significance of hydrogen bonding cannot be overstated. These bonds provide water with a high specific heat capacity, allowing it to store large amounts of energy without significant temperature changes. This property creates stable environments in lakes, oceans, and rivers, which are critical for aquatic life.
When the temperature drops, these hydrogen bonds begin to form a lattice structure as the water freezes. This process is called nucleation, leading to ice's unique crystalline formation. Ice's expansion upon freezing occurs because the lattice arrangement occupies more space than the liquid form. This is why ice floats; its lower density compared to liquid water is crucial for aquatic ecosystems, as it insulates bodies of water during colder months.
Unique Properties of Water
Water’s molecular structure imparts several unique properties:
- High Surface Tension: Thanks to hydrogen bonding, water has a high surface tension that allows small insects to walk on it without sinking.
- Solvent Capabilities: Water is often called the "universal solvent" because it can dissolve a wide array of substances. This characteristic is vital for life, helping nutrients travel within organisms.
- Thermal Regulation: The ability of water to absorb and retain heat supports climate stability, which in turn affects weather patterns.
These properties are not just laboratory phenomena; they have real-world applications. For example, when you drop ice into a drink, it cools the liquid without rapidly reducing its temperature due to the specific heat capacity of water.
"Water is the source of life, overcoming even the toughest elements. Its properties are like a master key, opening doors to various processes in nature and human applications.”
When looking at the freezing process of water, these unique molecular characteristics exemplify why water behaves differently and how it plays a crucial role not just in the freezing process but in the larger context of natural and artificial systems. Understanding the molecular structure of water provides insight into its freezing behavior and its broader implications in the environment and industry.
The Process of Freezing
Freezing is more than just a transition from liquid to solid; it’s a vital process with implications across various fields including science, nature, and even our daily lives. Understanding this whole shebang helps grasp wider phenomena such as climate patterns and food preservation. More importantly, grasping how freezing works can empower us to take better control over our environments and day-to-day activities, like cooking and preserving foods. The mechanics involved in freezing—how water freezes—opens up a world of possibilities, whether for a cook preparing a meal or a scientist researching climate impact.
Nucleation and Crystal Formation
Nucleation is the initial step in the freezing process and involves the formation of microscopic crystals within the liquid water. Think of it as sprouting seeds in the garden. These minute crystals serve as the backbone for snowflakes or ice. Nucleation can be influenced by factors such as temperature and impurities present in the water, much like how a gardener’s soil can affect seed growth. This is where the concept of temperature plays a significant role. When water cools below 0 degrees Celsius, it doesn't immediately turn to ice. Instead, it requires a seed—forming what we call nucleation.
There are two types of nucleation: homogeneous and heterogeneous. Homogeneous nucleation happens in pure water without impurities. It’s a rare occurrence because it requires a significant decline in temperature to create stable ice crystals. For most practical purposes, you’ll find that freezing mostly occurs via heterogeneous nucleation, which takes place in the presence of foreign particles. Consider how often we find ice with air bubbles or other debris trapped inside; this is a direct result of such nucleation.
Beyond simply forming crystals, these initial structures play a significant role in how the ice forms and how strong and expansive the ice becomes as it solidifies.
Energy Changes During Freezing
As water changes from a liquid to a solid state, energy changes are apparent and pivotal. Specifically, the process releases latent heat—a type of energy that doesn’t cause a temperature change but rather transforms the phase of the water. Picture a pot of boiling water. When you remove it from the heat source, it begins to cool but remains liquid until it reaches freezing point. Once it hits that point, something magical happens: it starts to release heat energy, transitioning smoothly into ice.
This heat release is why ice can often feel colder than anticipated, even if it's just resting in a freezer. The energy release during freezing can heavily depend on the environmental context, whether it's a closed freezer or an outdoor setting—temperature and air pressure factors into how efficiently energy is released. In understanding this, you realize energy changes are fundamental in assessing how freezing takes place in various setups and applications.
"The world of ice is not merely cold; it encapsulates complex energy shifts and intricate formations that define our planet's climates and ecosystems."
Environmental Influences on Freezing
The freezing process of water is not just a straightforward drop in temperature; it's a complex dance influenced by various environmental factors. Understanding how these influences shape freezing behavior helps us appreciate the delicate balance of nature. Atmospheric conditions, impurities in water, and surrounding elements contribute significantly to how ice forms, survives, and interacts with the ecosystem. In this section, we will delve into these influences in detail.
Impact of Atmospheric Conditions
Atmospheric pressure and temperature are two major players when it comes to freezing. High humidity levels can impact how ice forms on nearby surfaces. When the temperature dips, water vapor turns into ice crystals. This process can create frost on windows or even frostbite in more extreme conditions. The role of atmospheric pressure, in tandem, shouldn’t be overlooked. When pressure is low, the freezing point of water decreases, which means water might freeze at temperatures higher than the standard 0 degrees Celsius.
"Understanding the subtleties of atmospheric conditions can be the difference between a smooth ice layer and a snow-covered chaos".
For instance, think about a calm winter evening. If the air is still and humid, surfaces might develop a thin layer of ice due to condensation. On the flip side, on a windy day, moisture is whisked away, preventing freezing altogether. Additionally, altitude plays a role; at higher elevations, air is thinner and tends to be cooler, potentially leading to different ice formation behaviors compared to lower altitudes.
Effects of Temperature Variations
- Rapid Cooling: Quick drops in temperature can lead to sudden ice formation, which might catch you off guard, especially if you're outside.
- Gradual Cooling: When temperature falls slowly, ice can gradually form, allowing for better adherence to surfaces.
In creating an environment for ice formation, understanding local weather patterns, and keeping an eye on humidity levels can help predict freezing scenarios.
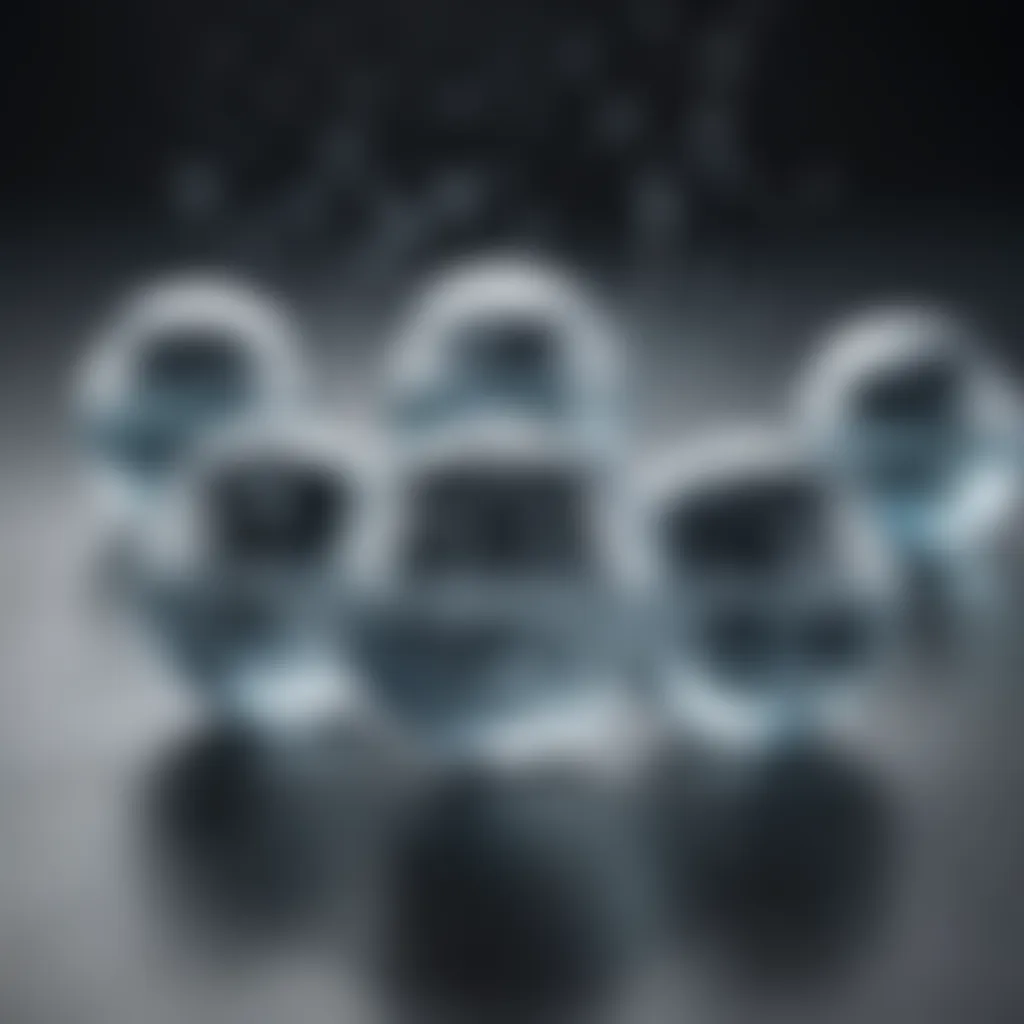
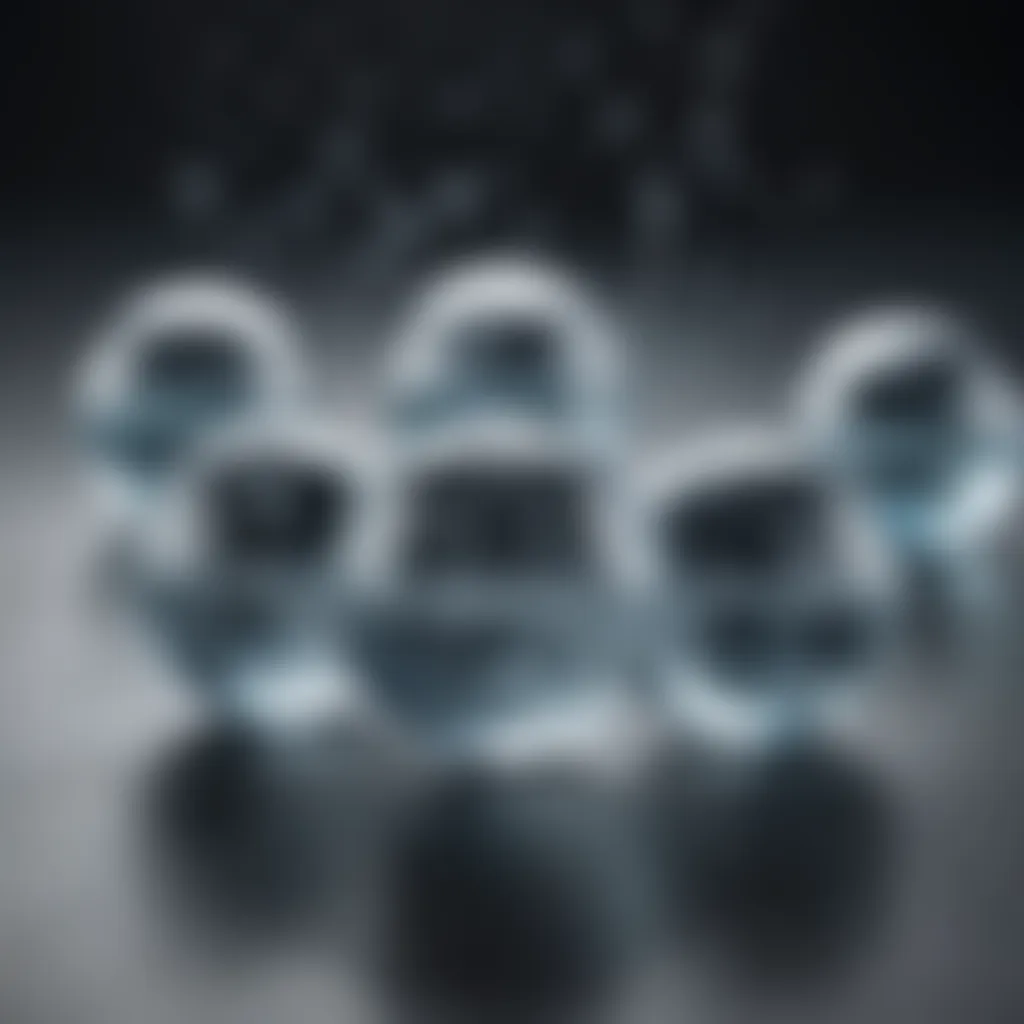
Influence of Impurities in Water
Water isn’t always pure, and those impurities can drastically influence the freezing point. For one, the presence of salts can lower the freezing point of water, a phenomenon widely known as freezing point depression. This is why salt is commonly spread on icy roads; it helps keep them clear in winter by preventing ice from forming — a clever way to manage hazardous conditions.
Consider how different types of water respond. For instance:
- Saltwater vs. Freshwater: Saltwater freezes at a lower temperature than freshwater, which explains why oceans rarely freeze, while lakes and ponds can become solid in winter.
- Contaminants: Even tiny particles like dirt or organic matter can affect ice formation. The more impurities present, the more energy is required for freezing, which affects the clarity and strength of the ice.
When discussing the effects of impurities, it might help to think of ice cubes you make at home. When you use tap water, sometimes the cubes are crystal clear, while other times they are cloudy due to dissolved minerals. This offers a tangible example; the cloudiness indicates impurities that disrupt neat crystal formation, making them weaker.
In summary, the environmental influences on freezing water surface not just physical but also chemical phenomena. By understanding these elements, we can harness the knowledge to both predict and manage freezing behaviors in various contexts, from ensuring safe outdoor conditions to controlling ice in industrial applications.
Applications of Water Freezing
Understanding the applications of water freezing can reveal its significance across various domains, showcasing its diverse benefits and necessary considerations. When water freezes, it doesn’t just create ice cubes for drinks. Instead, it plays an essential role in environmental systems, climate stabilization, industrial processes, and even culinary practices. The moment water transforms into ice, it initiates a cascade of changes. This transition is not just about lowering temperatures; it’s about influencing ecosystems and human lifestyles.
Impacts on Climate and Ecology
The freezing of water has profound implications for climate and ecological systems. Ice serves as a vital component in regulating the Earth’s temperature. It reflects sunlight, which helps to maintain cooler temperatures. Without this reflective surface, the absorption of solar energy would drastically accelerate warming, causing severe disruptions in weather patterns.
Moreover, ice formation in polar regions serves as a crucial habitat for various species. For instance, seals and polar bears rely on sea ice for breeding and hunting. Disruptions in the freezing season can impact their populations and overall ecosystem balance. According to research, significant climate changes causing premature melting can lead to decreased biodiversity and habitat loss.
"Ice is integral; it stabilizes the climate and supports countless species. Disruption may have far-reaching consequences beyond what we can see."
In thawing regions, even temporary surface ice affects local weather and temperature patterns. For example, frozen lakes release cold air that influences nearby climates, creating areas cooler than those that are ice-free. This interplay highlights the intricate connections between freezing processes and ecosystem dynamics.
Industrial Utilizations of Ice
Ice is a versatile matter with practical applications across industries, from food preservation to construction. In the food industry, ice is used extensively to preserve freshness and quality. Glistening ice packs ensure that perishable goods maintain their integrity during transport and storage, minimizing spoilage.
In construction, ice is utilized for its unique properties in various ways. For instance, engineers sometimes employ ice to create controlled environments for building, using chilled ice in portable refrigeration systems to maintain a specific atmosphere. This method can be beneficial in maintaining precise temperature requirements for certain materials.
Furthermore, ice plays a role in the chemical industry. When specific reactions require low temperatures, ice baths are used to control thermal conditions ensuring that products are synthesized effectively. Operating at optimal frozen conditions can influence yields and purity outcomes.
In summary, the applications of water freezing are far-reaching and significant. From preserving ecological balance to serving as a key element in myriad industrial processes, the frozen state of water is not just a simple occurrence. It is a fundamental process that underpins many aspects of our world. When we delve into these applications, we realize that the freezing of water is much more than just creating an ice cube in a drink; it's a vital process that sustains ecosystems and facilitates countless human activities.
The Phenomenon of Supercooling
Supercooling is an intriguing concept that showcases just how peculiar the freezing process of water can be. It happens when water drops below its normal freezing point of 0 degrees Celsius, yet remains in a liquid state. This occurrence is not just a curiosity; it has significant implications in both nature and various human endeavors.
Definition and Mechanisms
In essence, supercooling is when a liquid is cooled below its freezing point without solidifying. This anomaly arises because ice needs a seed, or crystal structure, to begin forming. Without any impurities or surface for nucleation, pure water can stay in a liquid state well below zero degrees Celsius. The conditions must be just right—often involving clean containers and undisturbed liquid.
Here’s how it works: when excessive cooling occurs, thermal agitation slows down but doesn't completely cease. Without the necessary triggers, water molecules refuse to organize into a solid arrangement. Some researchers even argue that liquids exhibit an innate tendency to resist transformation under specific conditions. The deeper you delve into the specifics, the more fascinating these interactions become.
Practical Implications of Supercooling
The practical implications of supercooling are significant in several fields. In meteorology, it's essential for understanding phenomena such as frost formation and the physics behind winter weather events. Similarly, in the realm of culinary applications, supercooled liquids can play a role in creating unique textures in food, leading to innovative culinary arts.
- Ice Cream Manufacturing:
- Cryopreservation:
- Sports and Leisure:
- Supercooling allows manufacturers to create smoother textures that reduce the formation of ice crystals, leading to creamier ice cream.
- Scientists utilize supercooling to preserve living cells, tissues, and even organs for transplantation. They can maintain biological samples in a state that delays decay while avoiding the damaging effects of forming ice crystals.
- In the world of winter sports, understanding supercooled water can influence the quality of ice rinks, enhancing conditions for athletes.
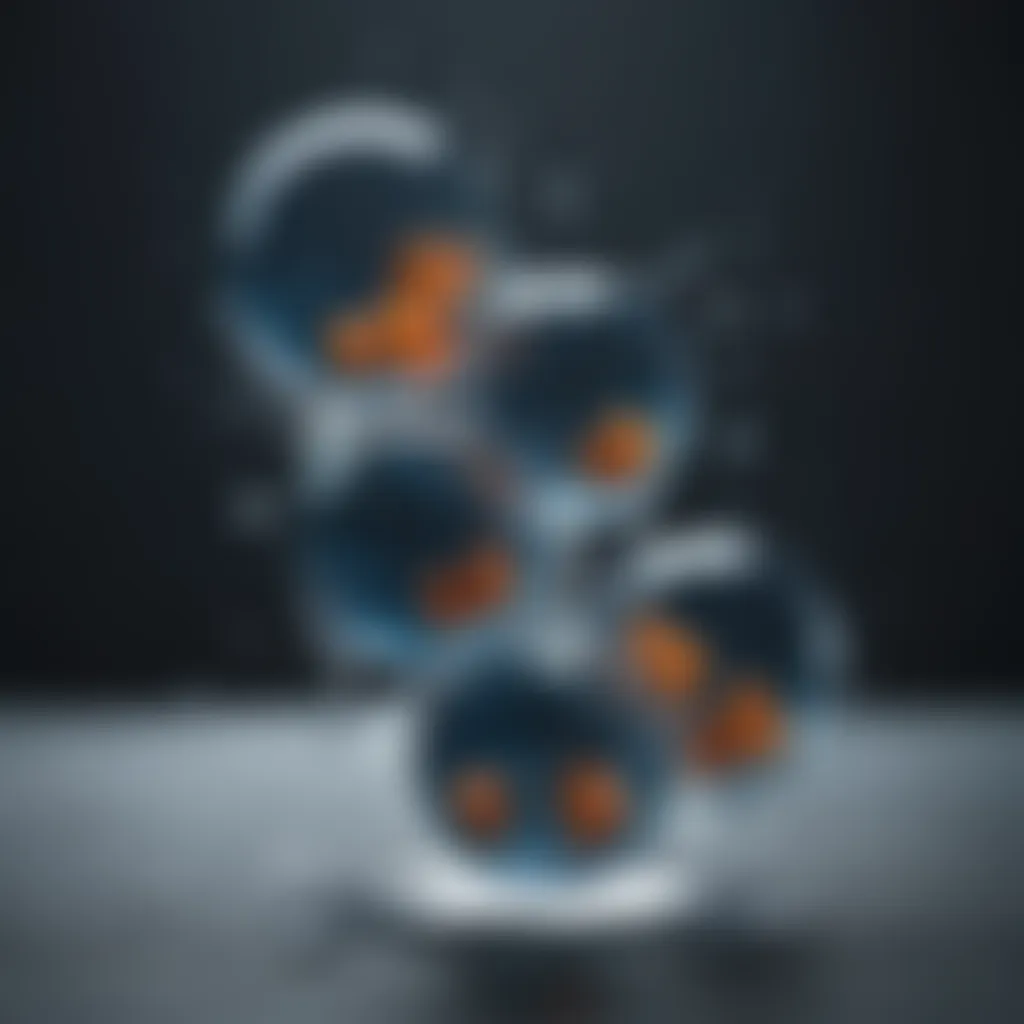
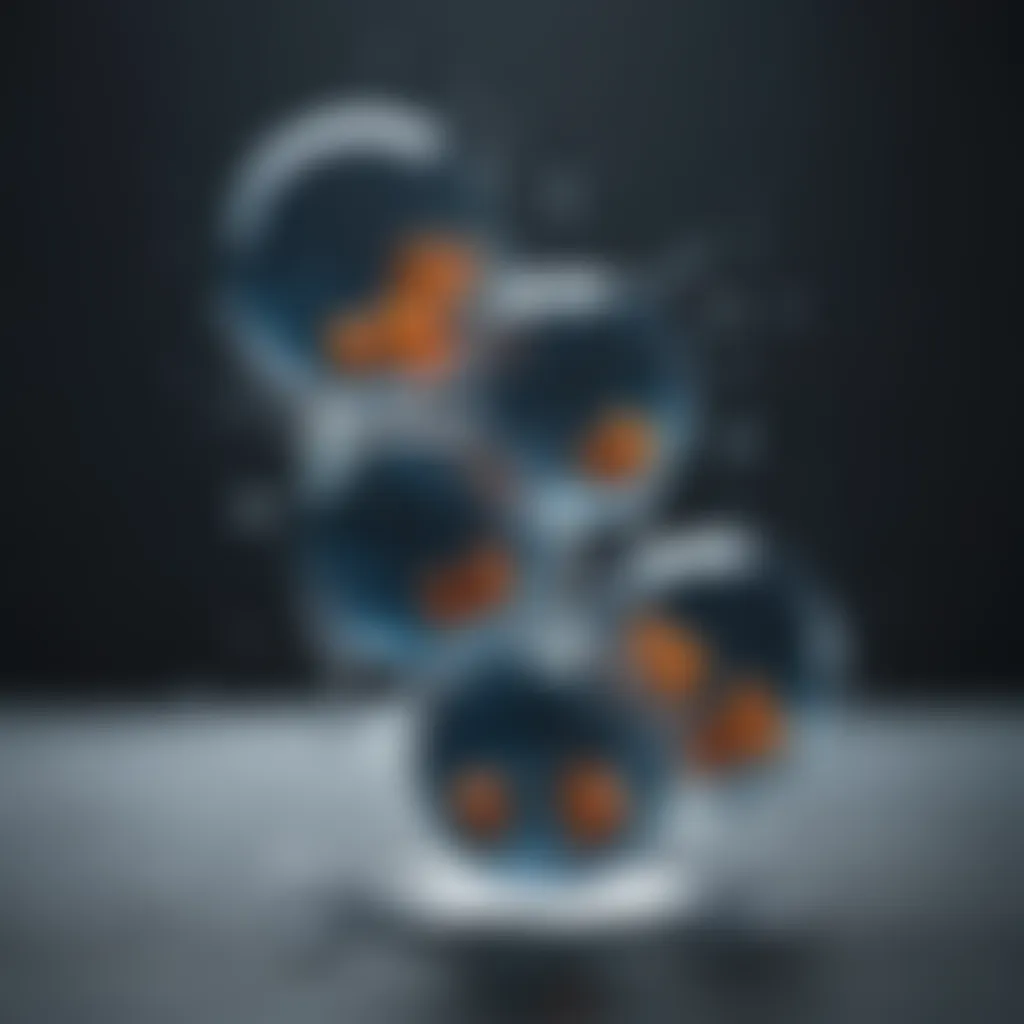
Supercooling showcases not only the wonders of physics but also the extraordinary versatility of water as a substance. With ongoing research in this area, who knows what other applications or effects might be uncovered in the future?
"In the world of supercooled water, mystery and science blend, hinting at deeper relationships between temperature, pressure, and phase changes."
Understanding this phenomenon is crucial. It opens avenues for innovations across different sectors while shining a light on the water's complex behavior, blending the lines between nature and technology.
Behavior of Ice in Different Environments
Understanding how ice behaves in various settings is like piecing together a beautiful quilt of nature's own design. Ice doesn’t just float or freeze; it interacts with its environment, showing us more than one facet of frozen water. This section will cover the subtleties of ice formation in natural bodies of water and the factors that affect its characteristics.
Ice Formation in Natural Bodies of Water
Ice formation is often painted as a simple process, yet its real-world implications are anything but. In natural water bodies like lakes or rivers, ice can form under a Aladdin's lamp of conditions. Factors such as temperature fluctuations, wind patterns, and even the presence of aquatic life can all contribute to how ice develops.
When water starts to cool, it contracts until it reaches about four degrees Celsius. After that, it begins to expand. This unique behavior causes ice to form on the surface first, rather than at the bottom, creating a layer that insulates the water below, allowing aquatic life to survive during frigid winters.
Moreover, the rate of ice formation isn’t uniform. During a still night, ice can thicken quickly, while in a windy setting, it may be much thinner due to disturbances at the surface. It is a dance of nature where each step has its own significance.
- Temperature plays a major role in forming ice:
- Wind is another element:
- Rapid cooling causes thicker ice.
- Gradual cooling can leave the ice more fragile.
- Covers the surface, creating waves.
- Can prevent the formation of thicker ice layers.
Effects of Salinity and Other Variables
The environment truly is a stage for ice, and the presence of variables like salinity can alter the play. In freshwater versus saltwater settings, the game changes dramatically. Salt lowers the freezing point of water, meaning that ice formation in seas or salt lakes is distinctly different from that in freshwater lakes.
When it comes to more intricate variables, think of pollution or sediment. These can act as obstacles to freezing in various ways:
- Altered freezing points:
- Salinity levels:
- Pollutants often disrupt the hydrogen bonding and can lead to ice forming at warmer temperatures or becoming less stable.
- Can lead to thinner, weaker ice structures in coastal areas, posing risks for marine life and even human activities like ice fishing.
"Ice is like a magician; it changes its form based on the elements but remains a solid presence in our lives."
Understanding these dynamics can help us fathom why some bodies of water freeze over more efficiently than others.
In essence, the behavior of ice—not just at a glance, but under a microscope of conditions—allows us to appreciate the complexity of nature's freezing process.
Future Research Directions
In the exploration of how water freezes, future research directions stand out as critical paths that can enhance our understanding and applications of this fundamental process. Investigating these directions not only promisingly illuminates the scientific landscape but also highlights the practical implications for both nature and industries that rely on water's unique properties. As we further unravel the techniques used and consider the universal environments that ice can be found in, we can anticipate breakthroughs that may impact climate studies, engineering practices, and many aspects of technology.
Advanced Ice Research Techniques
Research techniques in the study of ice have evolved significantly, integrating traditional methods with cutting-edge technology. Some of the advanced methodologies include:
- Cryo-Microscopy: This method allows scientists to capture microscopic images of ice crystals at ultra-low temperatures. It can help identify the formation processes and characteristics of ice at various stages.
- X-Ray Diffraction: Here, researchers utilize X-rays to analyze the atomic structure of ice. X-ray techniques can offer insights into the arrangement of water molecules, crucial for understanding ice's physical properties.
- Simulation Models: Computational models simulate various freezing scenarios, giving researchers a platform to predict how water behaves under distinct conditions without needing a laboratory for every experiment.
- Remote Sensing Technologies: Satellite imagery and geographical information systems help monitor ice formation and melting trends in real-time, particularly over large bodies of water like oceans and significant glaciers.
These techniques pave the way for a thorough understanding of the fundamental aspects of ice, guiding future research and its application across multiple disciplines.
Exploring Ice in Extraterrestrial Environments
The notion of ice forms and its freezing mechanisms extend beyond our planet, captivating astrobiologists and physicists alike. Scientific missions have unveiled water in various forms on celestial bodies like Europa, a moon of Jupiter, and Enceladus, one of Saturn's moons. Here’s what is particularly interesting about this direction:
- Implications for Life: Scientists inquire whether ice can harbor life, as it may provide a protective medium and necessary chemical interactions. Understanding the processes of freezing under alien conditions can provide insights into potential ecosystems.
- Climate Modeling of Other Worlds: Studying how ice exists and behaves in extraterrestrial environments helps inform models on climate changes both on Earth and in these other realms. Knowledge from such studies can contribute to more intricate predictions of weather patterns and climate dynamics here.
- Future Missions and Technological Advancement: Investment in missions to these icy worlds encourages the development of new technologies and ice-analytical tools that could even have terrestrial applications.
Researching ice formation in environments other than Earth bridges gaps in geology, astronomy, and environmental science, highlighting how interconnected our universe truly is.
"Understanding ice's role beyond our planet opens a window into possibilities of life and reveals how chemical processes operate across different environments."